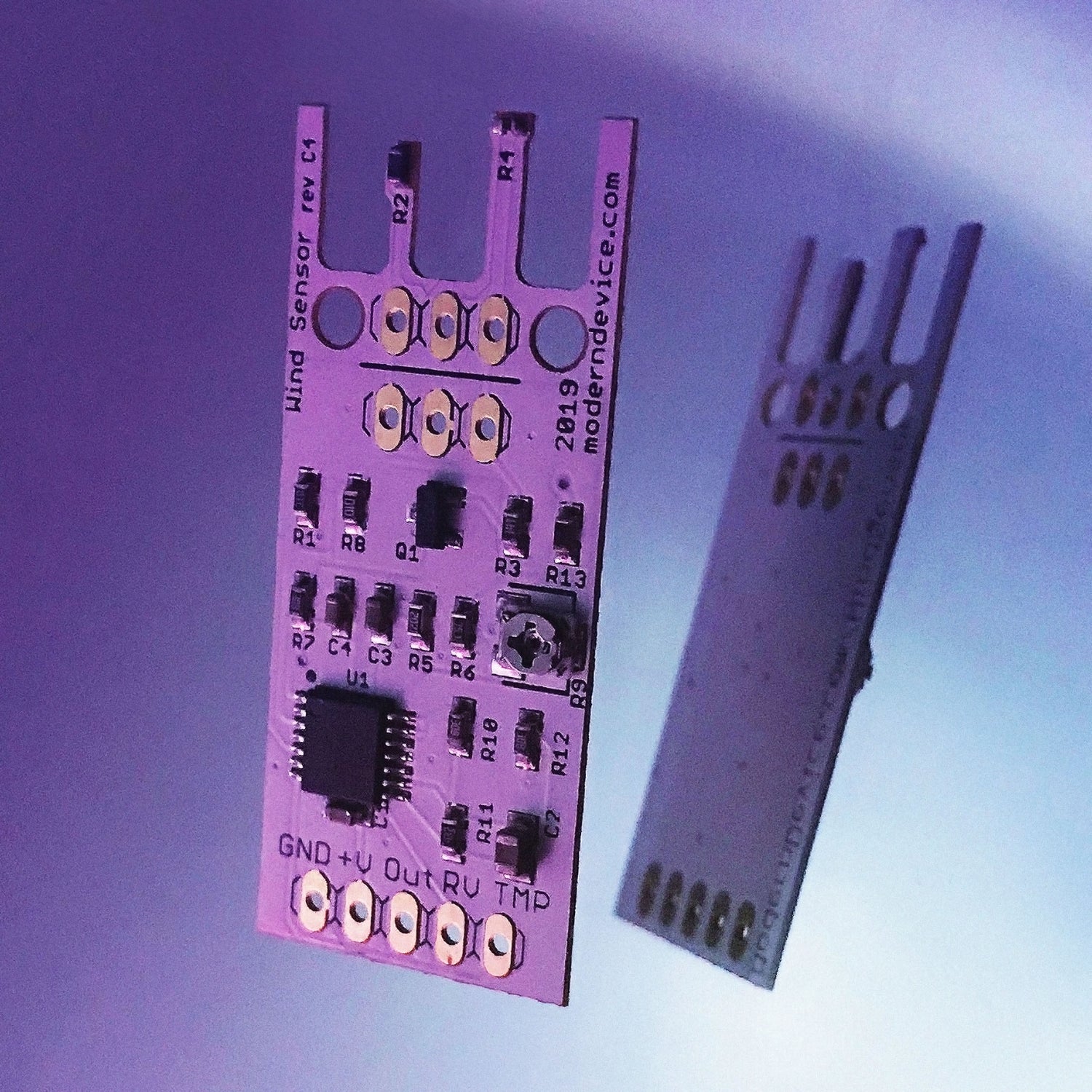
Precision Meets Innovation
Components for Industrial Applications and Creative Endeavors Alike. Serving Businesses and Makers Around the World.
Featured products
-
Wind Sensor Rev. C
Regular price $21.95Regular priceUnit price / per -
Wind Sensor Rev. P
Regular price $39.95Regular priceUnit price / per -
Reverbalizer: Hackable FV-1 Based Multi-effects Module
Regular price $59.95Regular priceUnit price / perSold out -
Sold out
Fluxamasynth Shield v.3
Regular price From $31.95Regular priceUnit price / perSold out -
Sold out
AC Current Sensor
Regular price $19.95Regular priceUnit price / perSold out -
Sold out
Pulse/Heartbeat Sensor
Regular price $21.95Regular priceUnit price / perSold out
1
/
of
6
Shop Collections
View all-
Synthesizers and Effects
Programmable synthesizers, sound generators, and effects, all in your control.
1
/
of
3